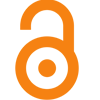
Research Article
Volume-1 Issue-1, 2022
Swelling of Nuclear Fuel in Nuclear Reactors
Received Date: June 14, 2022
Accepted Date: July 30, 2022
Published Date: Aug 01, 2022
Journal Information
Switch to Full Text Menu
Abstract
An attempt was made by inelastic scattering of nucleons on nuclei to elucidate the reasons for the excitation of nuclei in nuclear chain reactions, as well as the deformation of nuclei and the swelling of atoms. The proposed distorted-wave theory of multiple scattering of nucleons by nuclei is applied. When studying the properties of excited nuclei, the connections of the quadrupole vibrations of the nuclear surface with the motion of nucleons by the corresponding dipole and quadrupole giant resonances were taken into account. The energies spent on the excitation of giant dipole and quadrupole resonances with the corresponding energies and excitation widths, as well as on the vibrations of the nuclear surface, are determined. For a certain time, being in an excited state, the nucleus deforms and increases its size and the size of the atom, that is, its swelling.
Key words
Scattering of Nucleons, Excitation of Nuclei, Swelling of Nuclei and Atoms, Nuclear Reactors, Nuclear Fuel
Figure 1:Dependence of the double differential cross section at an incident proton energy of 800MeV, the scattering angle for a nucleus on the energy of scattered protons. The solid line is the results obtained, the dots are the experimental data [8] and the dotted line are the results obtained by the Glauber approximation method based on the Fermi gas model [9] |
Figure 2:Dependence of the differential cross section on the angle of scattering of protons on the nucleus at Ei = 800 MeV for dipole (L = 1) and quadruple (L = 2) giant resonances, as well as quadruple (? = 2) vibration of the nuclear surface. The solid line is the results obtained, the dots are the experimental data [8] and the dashed line denotes the results of the work calculated in the distorted-wave Born approximation [12] |
Background
Presently, applications of laser systems for material processing and surface treatments are increasing rapidly and gaining much interest, due to several advantages such as the speed, accuracy, and flexibility of this innovative technology. Today all organic, polymers like textiles and leather, can be treated and processed by laser radiation. Synthetic textiles are suitable for laser surface treatment, both for marking, engraving, and welding because they are thermoplastic, while, organic fabrics such as cotton, wool, and flax do not melt under the heat action of laser radiation.
Method
In order to clarify the reason for the excitations of nuclei, on the basis of quantum mechanics, in nuclear chain reactions, as well as the deformation of nuclei and the swelling of atoms, adhering to the well-known Bohr hypothesis about the independence of the decay of a compound nucleus from the method of its formation, we will consider the process of the appearance of highly excited states in target nuclei by scattering of nucleons.
The nature of highly excited states, giant resonances - monopole, dipole, quadruple, octuple and other resonances, which reflect more complex forms of coherent nuclear motion in the cross sections of nuclear reactions, which was explained within the framework of the semi classical hydrodynamic model of nuclei, was based on the concept of a single oscillation frequency of all neutrons nucleus relative to all of its protons in the interaction with the incident nucleon [5].
The nuclear reaction that occurs during inelastic scattering of nucleons of intermediate energies goes through the stage of formation of a “compound nucleus”. The strong interaction between the colliding nucleus and nucleons leads to the fact that very soon after the collision, the incident nucleon transfers a significant part of its energy to other nucleons of the nucleus. As a result of such a redistribution of energy, initially concentrated on one nucleon, not a single nucleon of the nucleus will have sufficient energy to overcome the action of the nuclear forces of attraction and leave the nucleus. This is the reason for the long lifetime of quasistationary states of excited nuclei. After some time, when, due to fluctuations, sufficient energy is concentrated on one of the nucleons (or on several nucleons), the compound nucleus will decay and possibly corresponding to different reaction channels [6].
In order to study this process, we write twice the differential cross section for inelastic scattering of nucleons, taking into account the width of the nuclear excitation energy, in the form of the Breit – Wigner formula [7].
here, Ef and Ef are the kinetic energies of the incident and scattered particles, (dσ/dΩ)NA is the nucleon-nucleon scattering cross section, in all nucleons of the nucleus. The momentum of the transfer of a nucleon to the nucleus by the target in this case takes the form
When studying the emerging giant resonances by inelastic scattering of nucleons, it is necessary to take into account the connection between oscillations of the nuclear surface and the motion of nucleons by the corresponding giant multipole resonances. Analysis of the structure of the low-energy spectra of spherical vibrational nuclei shows that the vibrations of the nuclear surface have a rather large amplitude and almost harmonic surface vibrations of the quadruple type [5]. Moreover, the value is of the order of 1MeV, while the energies of highly excited states of giant multipole resonances are greater than 15MeV. This means that at each moment of time, the oscillations corresponding to giant resonances occur, as it were, with a fixed shape of the nuclear surface. Therefore, in the dynamic collective model, it is necessary to take into account the interactions of giant resonance vibrations of nucleons with vibrations of the surface of the nucleus.
Results
The results obtained on the basis of theoretical calculations using the expression of the double differential cross section (1) for nucleons with energy MeV in comparison with the experimental and theoretical data calculated by the Glauber approximation method using the near Fermi gas model are shown in Fig.1.
From a comparison of the theoretical double cross section, depending on the energy of scattered protons, with experimental data [8], at the scattering angle, it follows that the energy loss of incident nucleons is ~ 45MeV. This energy is spent on the excitation of giant dipole and quadruple resonances with the corresponding energies and excitation widths: 18,131=Ω13,18 MeV and Γ13,21=ÃMeV; 18,212=Ω21,18 MeV and Γ2 1,42=ÃMeV, as well as on the vibration of the nuclear surface with energy - 09,42=ω 4,09 MeV and width Γλ=2 = 0,23MeV [9].
Thus, after determining the excitation energies of giant multipole resonances and the energy losses of scattering protons, one can begin to study the differential cross sections for the scattering angles. The angular dependences of the analyzing power are highly oscillating curves, the shape of which is largely determined by all nuclear parameters.
In the present paper, we have investigated the effect of the CO2 laser beam with different energies on the surface of five selected samples with the same thickness approximately of synthetic textiles. Due to their characteristics, synthetic textile materials are considered the better choice for treating by CO2 laser for marking, engraving, cutting, and welding processes.
The differential scattering cross section has the following form [10]:
The obtained results of calculations in dependence of the differential cross section on the angle of scattering of nucleons on the nucleus at 800MeV for dipole (L = 1) and quadruple (L = 2) giant resonances, as well as quadruple (λ = 2) vibration of the nuclear surface are presented in comparison with experimental data and theoretical calculations obtained in a distorted-wave Born approximation in Fig.2
The parameter characterizing the root-mean-square deformation of the excited nucleus is determined using the expression
for which, at the value of the stiffness parameter C2vib=224MeV, we have β=0,205.
Note that due to the lack of experimental work on the scattering of nucleons by radioactive nuclei, the calculations were performed for a lead nucleus.
Discussion
Thus, we can come to the conclusion that the excitation energy is spent on the excitation of giant dipole and quadrupole resonances with the corresponding energies and widths, as well as the surface excitation of the nucleus, which is in excited states for a certain time. At the same time, the core, being deformed, increases its size. This leads to an increase in the size of the atom, that is, its swelling, ultimately, and to the swelling of nuclear fuel.
References
- Qian Z, Liu W, Yu R, Tao Y, Yun D, Gu L. (2021) A kinetic rate theory modeling of fission gas release and fuel swelling for UN fuels. J Nuclear Materials. 556:1531-88.
- Zhang J, Wang H, Wei H, Zhang J, Tang C,et al. (2021). Modelling of effective irradiation swelling for inert matrix fuels. Nuclear Engineering and Technology. 538: 2616-28
- Baranov VY, Fizmatlit M (2005) Isotopes: properties, production, application. v.2, edited by p.115.
- Levin VE (1976) Nuclear physics and nuclear reactors. M Atomiz dat (1976)
- Aisenberg I, Greiner V (1975) Models of nuclei, collective and single-particle phenomena. M Atomizdat 325.
- Davydov AS (1958) Theory of the atomic nucleus. Fizmatgiz Moscow 611.
- Mirabutalybov MM (2014) Analytic Disorted-Wave Approximation for Intermadate Energy Proton Scattering Calculations. VIII Mezinarodni Vedecko-Practika Conference, 27 brezen-05 dubnaroku, Dil 83 Fyzika, Praha 3-7.
- Chrien RE (1980) Quasielastic scattering protons at 800 MeV. Phys Rev C 21: 1014-1019
- Esbensen H, Bertsch GF (1986) Quasielastic proton-nucleus scattering at 300-800 MeV. Phys Rev C Nucl Phys 34: 4.
- Mirabutalybov MM (2010) Excitation of giant resonances in nuclei by inelastic scattering of protons. Proceedings of Higher Educational Institutions. Physics MHE Institutions. Russ 3: 59-73
- Bertrand FE, Gross EE (1986) Giant resonance structure in measured using the reaction at 334 MeV Phys. Rev C Nucl Phys 34: 45-55.
- Adams GS, Cook D (1986) Study on in medium nucleon-nuclear cross sections. Phys Rev C 33: 2054-2058.
Artcle Information
Short Communication
Received Date: June 14, 2022
Accepted Date: July 30, 2022
Published Date: Aug 01, 2022
Journal of Energy and Renewable Resources
Volume 1 | Issue 1
Citation
Mirteimour MM (2022) Swelling of Nuclear Fuel in Nuclear Reactors. J Energy Renewable Resour 1: 1-5
Copyright
©2022 Mirteimour MM. This is an open-access article distributed under the terms of the Creative Commons Attribution License, which permits unrestricted use, distribution, and reproduction in any medium, provided the original author and source are credited.
doi: jerr.2022.1.104